Sodium-Potassium pump
Na+/K+-ATPase (also known as the Na+/K+ pump, sodium-potassium pump, or simply NAKA, for short) is an enzyme (EC 3.6.3.9) located in...
Northern blot
Northern blot is a technique used in molecular biology research to study gene expression. It takes its name from its similarity...
Lynch Syndrome
Hereditary nonpolyposis colorectal cancer (HNPCC), also known as Lynch syndrome, is characterised by a risk of colorectal cancer and other cancers...
Nano-BioEngineering
.jpg)
Biomarker discovery is the process by which biomarkers are discovered. It is a medical term. Many commonly used blood tests in...
Role of Enzymes
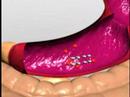
Enzymes serve a wide variety of functions inside living organisms. They are indispensable for signal transduction and cell regulation, often via...
Lecture on Pharmacogenomics and Cancer

Pharmacogenomics is the branch of pharmacology which deals with the influence of genetic variation on drug response in patients by correlating...
Organelle Movement on Microtubules
This video deomonsrates the movement of organlles on microtubules. ...
Transfer RNA (tRNA)
Transfer RNA (abbreviated tRNA) is a small RNA molecule (usually about 74-95 nucleotides) that transfers a specific active amino acid to...
Atherosclerosis and Ischaemic Stroke
Atherosclerosis is a disease affecting arterial blood vessels. It is a chronic inflammatory response in the walls of arteries, in large...
Nanowires and Nanocrystals for Nanotechnology
Yi Cui is an assistant professor in the Materials Science and Engineering Department at Stanford University. He is a recipient of...
Using p53 to Fight Cancer
Adenovirus infects all the cells ,In normal cell to DNA from the virus moves towards to the nucleus which has a...
Immune system Protection Against Cancer
Tumor cells have developed mechanism to avoid dectection by immune system,when Left undetected Tumor cells will continue to grow and progress.However...
MacroPhage in Immune system
Macrophages are cells within the tissues that originate from specific white blood cells called monocytes. Monocytes and macrophages are phagocytes, acting...
Nanobiotechnology:putting Molecular Machines to work
Describes in general terms the concepts of high-throughput protein expression coupled with immobilizations in functionalized nanoporous materials to carry out multiple...
Mechanical Engineering in Bioengineering
My general research interests lie in elucidating the inner-workings of proteins, enzymes and biological motors, using instrumentation that combines optical tweezers,...
Endothelial keratoplasty surgery.
Lamellar keratoplasty involves replacement of your damaged or diseased anterior corneal stroma (middle layer of your cornea) and Bowman's membrane (second...
Meniscus Injury Video
Meniscus is a crescent-shaped fibrocartilaginous structure present in the knee, acromioclavicular, sternoclavicular, and temporomandibular joints that, in contrast to articular disks,...
Targets for Cancer, Crohn's disease, ALS
Cancer (medical term: malignant neoplasm) is a class of diseases in which a group of cells display the traits of uncontrolled...
How Does an Egg Make an Organism
Lecture is presented by Sir John Bertrand Gurdon ,In 1962, Gurdon, then at Oxford University, announced that he had used the...
Proteasomes Animation
Proteasomes are large protein complexes inside all eukaryotes and archaea, as well as in some bacteria. In eukaryotes, they are located...
Anti-Angiogenesis as Strategy for Cancer Inhibition
The tocotrienol (or TCT) group—together with tocopherols—compose the vitamin E family. Natural tocotrienols exist in four different forms or isomers, named...
Follicular phase
The follicular phase (or proliferative phase) is the phase of the estrous cycle, (or, in humans and great apes, the menstrual...